Imaging Electro-mechanical Waves
The measurement of electro-mechanical waves in cardiac tissue remains a major experimental challenge. Conventional fluorescence imaging of the heart (optical mapping) is significantly compromised by tissue deformation resulting in substantial motion artifacts in the optical signal. This limitation is usually addressed by preventing contractile motion using pharmacological motion uncouplers, which are known to alter the dynamical properties of the tissue. To overcome these limitations, we have developed an advanced optical mapping system that is capable of imaging contracting tissue. Using innovative camera calibration and image registration techniques, we not only recover the undisturbed fluorescence signal (i.e. membrane potential and/or intracellular calcium), but also obtain surface displacement and strain. However, with excitation and emission wavelengths in the visible or near infrared, optical mapping does not permit to measure waves inside the heart wall. Therefore, we have proposed a new imaging modality based on ultrasound elastography [2,3], which shows promise to visualize patterns of propagation of excitation waves inside the cardiac tissue through the mechanical deformations they induce, as shown in Fig. 1. We have provided a numerical proof-of-concept of this novel imaging modality, indicating the potential for novel non-invasive structural and functional diagnosis of the heart.
Figure 1
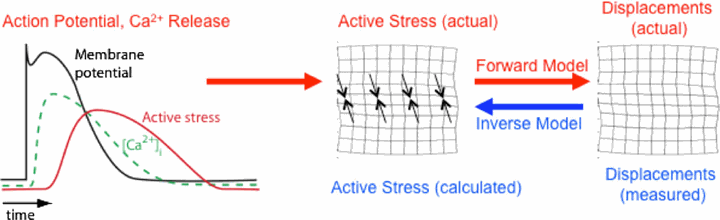
As indicated by the red arrows, the passage of action potentials through individual cells causes time-dependent processes in these cells (left panel), which lead to local contraction of the tissue primarily in the fiber direction (black arrows in the center panel; with the large-scale tissue represented schematically as an imaginary rectangular grid drawn on an arbitrary cross section of the tissue), causing deformation and therefore displacement of the entire tissue (right panel). Computer models of both of the forward problem (the creation of displacements from known active stresses; red arrow on right) and the inverse problem (the determination of the stresses that had to be present to create a given displacement field; blue arrow) are used in this study (from [1]).
In order to advance this new diagnostic modality, we study the propagation of electrical and mechanical waves in engineered heart tissue (EHT). EHT is available in the form of ring-like artificial tissue constructs with quasi-physiological action potential propagation and force development [4]. EHT are made in the Department of Pharmacology (University Medicine Göttingen, UMG). Due to their simple, accessible geometry, they are an ideal “test bed” for the development and evaluation of novel imaging modalities as well as quantitative, data driven modeling of cardiac electro-mechanics. Mechanical motion and electrical activation in EHT is observed simultaneously using high-resolution ultrasound (Vevo 2100, VisualSonics Inc.) and fluorescence imaging using calcium and voltage sensitive dyes (Fig. 2A). Mechanical motion obtained from the local displacement of the ultrasound speckle pattern using a cross-correlation technique. To advance the development of a three-dimensional ultrasound imaging modality, we studied the propagation of periodic wave propagation by stroboscopic ultrasound imaging in transversally shifted image planes (Fig. 2B) The resulting three-dimensional, spatio-temporal data set reveals three-dimensional wave propagation and characteristic filament-like strain patterns (Fig. 2C). These strain patterns are observed in the vicinity of muscle fibers, as evidenced by confocal microscopy. Further experiments are underway to characterize the structural and functional development of EHT during maturation. Our experimental findings suggest that the available spatial and temporal resolution should permit the observation of transient phenomena.
Figure 2
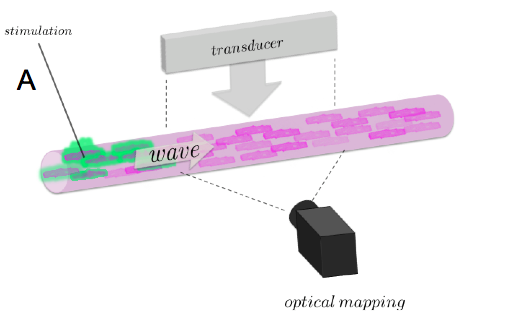
(A) Experimental setup for studying electromechanical waves in EHT constructs showing high-resolution ultrasound transducer and its imaging plane (Vevo 2100, VisualSonics Inc.), fluorescence imaging camera (MiCAM Ultima L, SciMedia Inc.), and stimulation electrode.

(B) Three-dimensional elastography using parallel imaging planes with periodic stimuli.

(C) Qualitative comparison of strain pattern (red iso-surface) with muscular fiber structure obtained using macroconfocal microscopy. Red points within the EHT indicates actin and muscle tissue. The 3D strain pattern shows a magnification of a small, representative volume within the EHT (dashed lines).
References
- F.H. Fenton and A. Karma, Chaos 8, 20-47 (1998).
- N.F. Otani, S. Luther, R. Singh and R.F. Gilmour, Ann. of Biomed. Eng. 38, 3112-3123 (2010).
- N.F. Otani et al., Computers in Cardiology 36, 617-620 (2009).
- W.-H. Zimmermann et al., Circ. 106, I151-7 (2002).